Cosmic Microwave Background
The Echo of the Big Bang
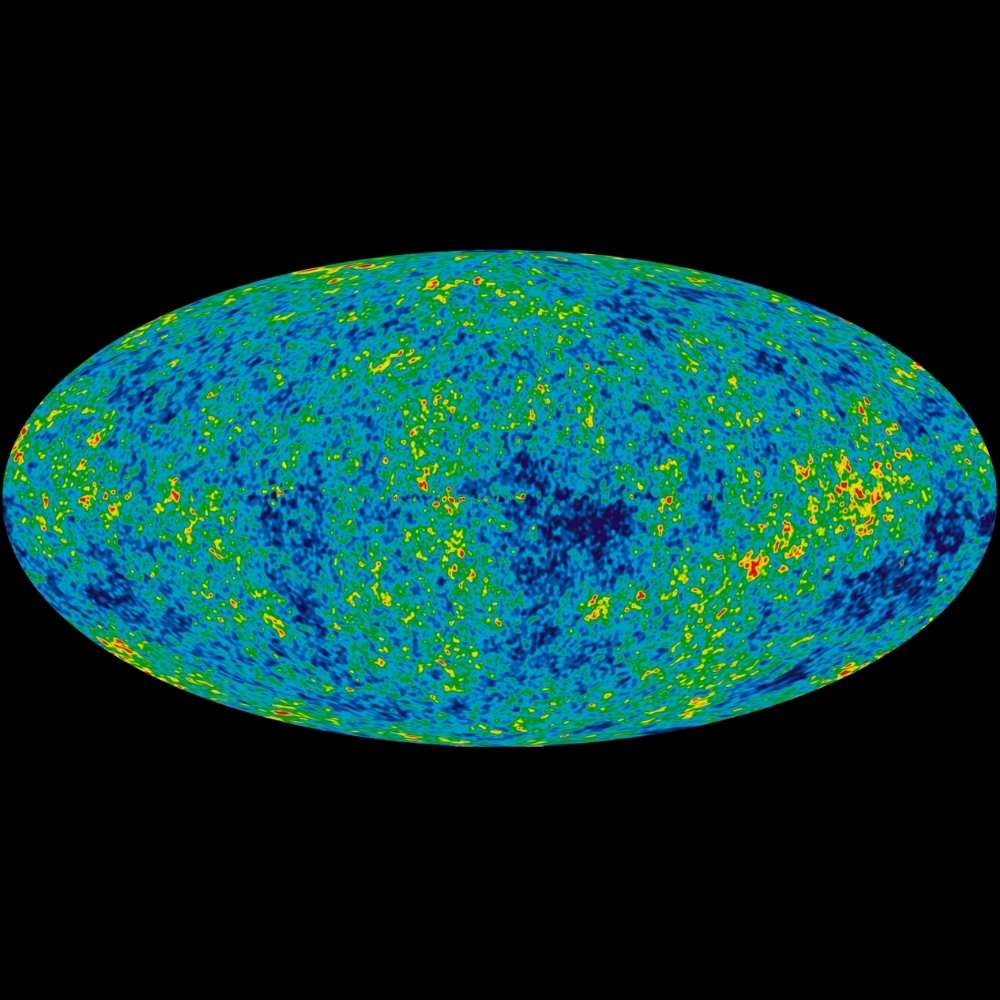
Quick Reader
Attribute | Details |
---|---|
Name | Cosmic Microwave Background (CMB) |
Type | Relic radiation (electromagnetic radiation) |
Discovered | 1965 by Arno Penzias and Robert Wilson |
Temperature | ~2.725 K (Kelvin) |
Peak Wavelength | ~1.9 mm (Microwave region) |
Age | ~13.8 billion years |
Origin | Recombination era (~380,000 years after the Big Bang) |
Observation Instruments | COBE, WMAP, Planck satellite |
Spectrum Type | Nearly perfect blackbody |
Significance | Strongest evidence for the Big Bang theory |
Anisotropies | Tiny temperature fluctuations indicating matter distribution in early universe |
Uniformity | Extremely uniform with variations ~1 part in 100,000 |
Observation Technique | Radio/microwave telescopes and satellites above Earth's atmosphere |
Related Concepts | Big Bang, inflation, baryon acoustic oscillations, large-scale structure |
Introduction – Light from the Dawn of Time
When we look into the deep night sky, we’re not just seeing stars — we’re seeing the past. But beyond the most distant galaxies, there exists something even more ancient: the Cosmic Microwave Background (CMB). This is not light from stars or galaxies — this is light from the very beginning of the universe, released when the cosmos was just 380,000 years old.
The CMB is the afterglow of the Big Bang — a faint whisper of ancient photons stretched into microwaves by the expansion of the universe. Its discovery was a scientific milestone that forever changed our understanding of the cosmos.
The Discovery of the CMB – A Serendipitous Revolution
In 1965, two Bell Labs scientists — Arno Penzias and Robert Wilson — were testing a radio antenna when they encountered a persistent noise. No matter where they pointed the instrument, the faint microwave signal remained. Unknown to them, this “noise” was the long-sought evidence predicted by Big Bang cosmologists.
Their discovery, made unintentionally, confirmed theoretical predictions from the 1940s that the universe must be filled with relic radiation from its hot, dense past.
Key Timeline:
1948 – George Gamow and colleagues predict relic radiation.
1965 – Penzias and Wilson detect it.
1978 – They win the Nobel Prize in Physics.
1992 – NASA’s COBE satellite detects anisotropies in the CMB.
2003–2013 – WMAP and Planck map the CMB in exquisite detail.
What Created the CMB?
In the early universe, everything was an opaque, hot plasma — light couldn’t travel freely. But as the universe expanded and cooled, protons and electrons combined to form neutral hydrogen in an event called recombination. At this moment:
The universe became transparent to light.
Photons began to travel freely.
This light is what we detect today as the CMB.
That light has cooled due to the universe’s expansion, shifting from visible/infrared to the microwave region of the electromagnetic spectrum.
Properties of the CMB – A Perfect Snapshot of the Young Universe
Temperature: The CMB is astonishingly cold today — about 2.725 K, just above absolute zero.
Uniformity: It is remarkably smooth in all directions, but with tiny fluctuations.
Anisotropies: These tiny fluctuations (~0.00001 K) reveal the seeds of galaxy formation.
Spectrum: The CMB has a near-perfect blackbody spectrum, making it one of the most precise measurements in cosmology.
The CMB Anisotropies – Seeds of Galaxies in a Silent Map
While the CMB appears nearly uniform, high-resolution measurements reveal minute temperature fluctuations. These anisotropies are not noise — they are imprints of the early universe’s density variations, frozen into the radiation.
Why Are These Fluctuations Important?
Regions slightly denser than average had stronger gravitational pull.
These areas later attracted more matter, forming galaxies and clusters.
Less dense regions became cosmic voids.
In essence, the CMB map is like a baby photo of the universe — showing the tiny wrinkles that would grow into the entire cosmic web.
Visualization:
The well-known Planck and WMAP sky maps color-code temperature variations — red for slightly warmer regions, blue for cooler ones — on the microkelvin scale.
How Satellites Like COBE, WMAP, and Planck Changed Everything
COBE (1989–1993)
First to detect temperature fluctuations in the CMB.
Confirmed the blackbody spectrum of the CMB with extreme precision.
WMAP (2001–2010)
Mapped the anisotropies across the entire sky with far better resolution.
Determined key cosmological parameters:
Universe age: ~13.77 billion years
Matter content: ~4.6% normal, ~24% dark matter, ~71.4% dark energy
Flatness: Universe is geometrically flat
Planck (2009–2013)
Most detailed full-sky map of the CMB to date.
Detected temperature variations down to a few millionths of a degree.
Provided compelling support for inflation, a rapid expansion right after the Big Bang.
CMB Polarization – A New Window into Inflation
What Is CMB Polarization?
As CMB photons scattered off electrons during recombination, polarization patterns were imprinted. These patterns contain clues about the very first moments of the universe.
There are two types:
E-modes: Even, radial patterns — primarily from density variations.
B-modes: Swirly, curl-like patterns — potential evidence of primordial gravitational waves from inflation.
Why It Matters:
Detecting B-modes would confirm inflation theory.
BICEP2 (2014) initially claimed discovery, but dust contamination was later found.
Current and future missions (e.g., LiteBIRD, CMB-S4) aim to definitively detect these signals.
The CMB and the Shape of the Universe
Measurements of the CMB help answer profound questions like:
Is the universe flat?
→ Yes. CMB observations strongly indicate a spatially flat universe, where parallel lines stay parallel.How old is the universe?
→ About 13.8 billion years, based on the expansion rate (Hubble constant) and CMB spectrum.How much matter and dark energy is there?
→ The CMB tells us that:~5% is ordinary (baryonic) matter
~27% is dark matter
~68% is dark energy
A Sound Map – Baryon Acoustic Oscillations
In the early plasma, sound waves traveled through matter and radiation, creating pressure peaks. These oscillations left visible imprints in the CMB — known as baryon acoustic oscillations (BAO).
Later, these same ripples became:
Patterns in galaxy clustering
A “standard ruler” to measure cosmic expansion
Frequently Asked Questions (FAQ)
Q: Why is the Cosmic Microwave Background important?
A: The CMB is the strongest observational evidence for the Big Bang. It gives us a snapshot of the universe just 380,000 years after its birth, revealing the seeds of all structure we see today — galaxies, clusters, and cosmic voids.
Q: Can we see the CMB with our eyes or telescopes?
A: No. The CMB is microwave radiation, which lies outside the visible spectrum. It is invisible to optical telescopes and human eyes, but detectable with radio and microwave instruments like those on the Planck and WMAP satellites.
Q: Why is the CMB so cold?
A: When the universe first emitted the CMB, it was ~3000 K. But over 13.8 billion years, as the universe expanded, the light was stretched (redshifted) into microwaves, cooling it down to ~2.725 K today.
Q: Is the CMB the oldest light in the universe?
A: Yes. It’s the oldest detectable electromagnetic radiation, originating when atoms first formed and the universe became transparent to photons.
Q: What does the smoothness of the CMB tell us?
A: It reveals that the early universe was remarkably homogeneous, but the tiny fluctuations (anisotropies) gave rise to all modern cosmic structures. This supports theories like inflation and dark matter-driven formation.
Final Thoughts – Why the CMB Is a Pillar of Modern Cosmology
The Cosmic Microwave Background is not just ancient light — it’s a cosmic Rosetta Stone. Encoded in its gentle glow is the story of the early universe, the nature of matter and energy, and the fate of cosmic evolution.
Thanks to missions like COBE, WMAP, and Planck, we now understand:
The age and composition of the universe
That the universe is flat and expanding
That inflation likely shaped the cosmos before the CMB even formed
The CMB remains a foundational dataset for all future cosmology. New observatories — from ground-based microwave arrays to next-gen satellites — are poised to explore even finer details. The quest continues to detect primordial gravitational waves, trace neutrino imprints, and decode the earliest whispers of the Big Bang.