J0313-1806 (Most Distant Known Quasar)
Explore J0313–1806, the most distant known quasar from the early universe, with a 1.6 billion solar mass black hole just 670 million years after the Big Bang.
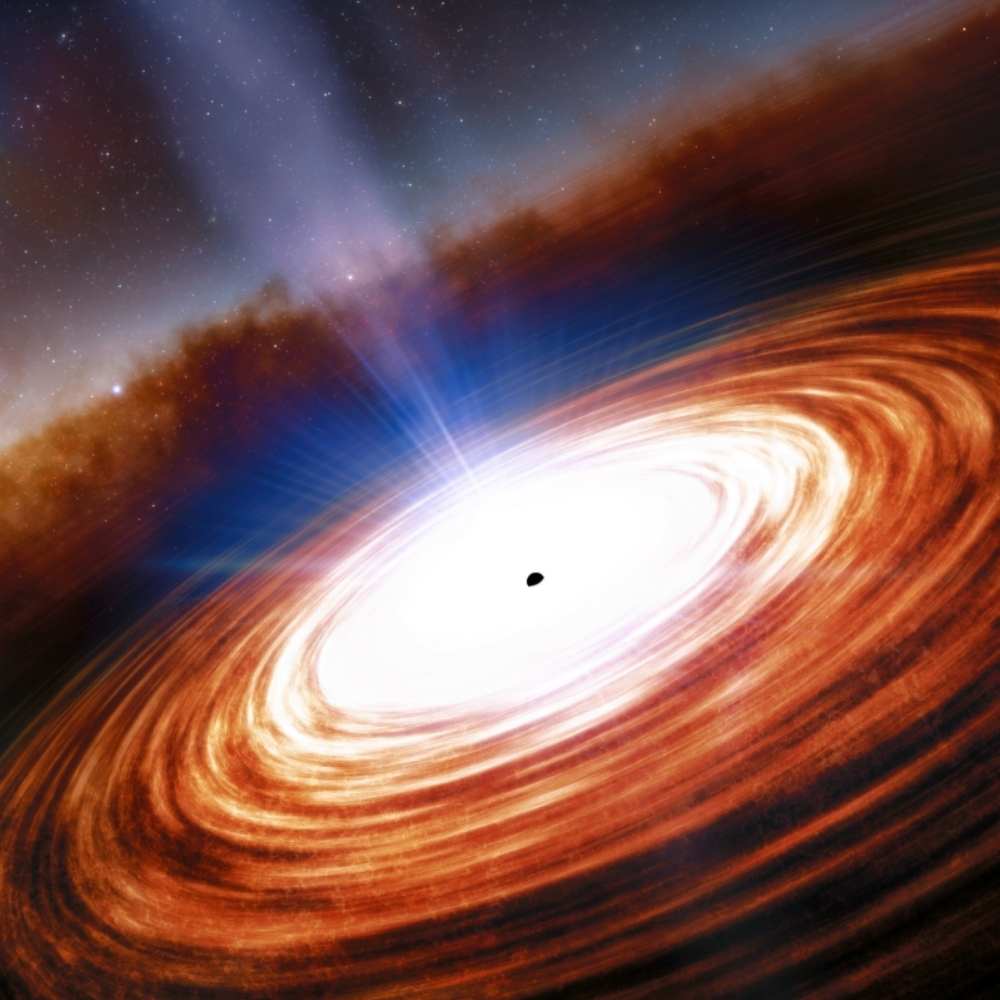
Quick Reader
Attribute | Details |
---|---|
Name | J0313–1806 |
Object Type | Quasar (Active Galactic Nucleus) |
Redshift (z) | 7.64 |
Light Travel Time | ~13.03 billion years |
Discovered In | 2021 |
Telescope Used | Maunakea Observatories (Hawaii) |
Black Hole Mass | ~1.6 billion solar masses |
Host Galaxy | Star-forming, high mass, powerful outflows |
Significance | Most distant confirmed quasar with massive black hole |
Observational Challenge | Faint, redshifted light in early universe |
Relevance | Challenges black hole growth models, traces reionization epoch |
Introduction – A Quasar from the Early Cosmos
Quasars are among the brightest and most energetic objects in the universe, powered by supermassive black holes actively consuming matter. They shine so intensely that they can outshine their entire host galaxy—and can be seen across billions of light-years.
In 2021, astronomers announced the discovery of J0313–1806, the most distant known quasar ever confirmed. With a redshift of z = 7.64, this quasar dates back to just 670 million years after the Big Bang, when the universe was less than 5% of its current age.
Even more surprising—at its heart lies a black hole with a mass of 1.6 billion times that of the Sun, already fully formed at a time when the first galaxies were just beginning to emerge.
What Makes J0313–1806 So Extraordinary?
This object isn’t just remarkable because of how far away it is—it also forces scientists to rethink how black holes grow.
Distance: At a redshift of 7.64, J0313–1806 is the most distant quasar with confirmed mass measurement.
Age: We observe it as it was only 670 million years after the Big Bang.
Black Hole Mass: At 1.6 billion solar masses, it is too massive to have grown by standard accretion models in such a short cosmic time.
Growth Implications: Suggests formation from a direct collapse black hole or other rapid mechanisms.
These findings imply that supermassive black holes formed much faster than previously thought—possibly bypassing the slow stellar-collapse route altogether.
How Was It Detected?
Discovering quasars at such extreme distances is a challenge. Their visible light is redshifted into the infrared spectrum, requiring specialized instruments.
J0313–1806 was detected using:
Pan-STARRS1 (PS1) and DECaLS surveys to identify high-redshift candidates
Gemini North and Keck I telescopes for spectroscopic confirmation
Infrared imaging to study host galaxy properties
The object’s spectral signature shows broad emission lines characteristic of an active galactic nucleus (AGN), confirming its identity as a quasar.
Host Galaxy and Outflows
Interestingly, the quasar’s host galaxy appears to be a massive, dusty, star-forming system, with clear evidence of:
Fast gas outflows (up to 20% the speed of light)
Active star formation
Heavy elements (metals) already present
These outflows are driven by the black hole’s feedback, and may eventually shut down star formation in the host galaxy—part of the galaxy–AGN co-evolution process.
Comparison with Other High-Redshift Quasars
Before the discovery of J0313–1806, the record-holder for the most distant quasar was ULAS J1342+0928, with a redshift of z = 7.54. That’s just slightly closer to us than J0313–1806, yet its black hole mass was already estimated at ~800 million solar masses—which was itself difficult to explain.
J0313–1806 not only broke the distance record, but also doubled the black hole mass—to 1.6 billion solar masses—at an even earlier epoch.
Quasar Name | Redshift (z) | Black Hole Mass | Age of Universe at Emission |
---|---|---|---|
J0313–1806 | 7.64 | ~1.6 billion solar masses | ~670 million years |
J1342+0928 | 7.54 | ~800 million solar masses | ~690 million years |
J1120+0641 | 7.08 | ~2 billion solar masses | ~750 million years |
This creates a paradox: how can such massive black holes form so early, when galaxies themselves were still assembling?
Black Hole Formation and Growth Models Under Pressure
The standard models of black hole growth begin with the death of Population III stars, the first stars in the universe. These stars may leave behind stellar-mass black holes (5–100 solar masses), which slowly grow by accreting gas or merging with other black holes.
But that growth is limited by the Eddington limit—a balance between the pull of gravity and the outward pressure of radiation. Even under optimal (Eddington-limited) conditions, it would take hundreds of millions of years for a black hole to grow to even a billion solar masses.
J0313–1806 appears too early and too massive for that timeline.
Alternative explanations include:
Direct Collapse Black Holes (DCBHs): Massive gas clouds collapse directly into black holes of ~10⁴–10⁵ solar masses, skipping the stellar phase.
Super-Eddington Accretion: Temporary violation of the Eddington limit may allow rapid early growth.
Primordial Black Holes: Formed directly from density fluctuations in the early universe (still speculative).
None of these mechanisms are fully proven—yet discoveries like J0313–1806 make their investigation increasingly urgent.
Insights into Early Galaxy Evolution
Because quasars like J0313–1806 are so luminous, they act as cosmic lighthouses, illuminating not only themselves but the space around them. Spectroscopy of these regions reveals:
Metallicity in surrounding gas
Presence of molecular clouds and dust
Signs of early galaxy feedback and regulation
J0313–1806’s host galaxy already shows mature characteristics—including enriched gas and high star formation—despite being less than 700 million years old. This suggests that galaxy–AGN coevolution began very early, reshaping the standard model where galaxies form first and black holes grow later.
A Beacon in the Reionization Era
J0313–1806 also serves as a valuable probe of the cosmic reionization epoch. The intense radiation from quasars contributes to ionizing the neutral hydrogen that filled the early universe. Studying the intergalactic medium (IGM) along the line of sight to J0313–1806 helps:
Trace the progress of reionization
Map the distribution of neutral vs. ionized gas
Measure Lyman-alpha absorption features in high-redshift environments
This makes distant quasars critical tools for understanding the last major phase transition in cosmic history.
Observational Challenges – Seeing the First Quasars
Detecting quasars like J0313–1806 requires overcoming several obstacles:
Extreme faintness: At such vast distances, even the brightest quasars are faint and redshifted into the infrared.
Foreground contamination: Low-redshift galaxies or cool stars can mimic the photometric signatures of high-redshift quasars.
Spectroscopic confirmation: High-resolution infrared spectroscopy is essential to validate redshift and identify emission lines like Lyman-alpha, Mg II, and C IV.
This level of precision demands large telescopes, advanced instruments, and long integration times—a combination available only at premier observatories such as:
Keck I and Gemini North (Hawaii)
Very Large Telescope (VLT, Chile)
James Webb Space Telescope (JWST)
Each discovery relies on an efficient multi-step process: first photometric identification, then spectroscopic follow-up, and finally deep imaging of the quasar’s host galaxy and environment.
JWST and the Hunt for Earlier Quasars
With its infrared sensitivity and spatial resolution, JWST opens an entirely new era in quasar discovery.
Expected breakthroughs include:
Detection of quasars at z = 9–11, formed within the first 400 million years
High-precision spectroscopy of known high-z AGNs
Imaging the host galaxies of early quasars in detail
Identifying black hole seeds and tracing their growth
JWST’s data will also help answer critical questions:
Were early quasars common or rare?
What are the environments of the first AGNs?
How are early black hole mass, galaxy mass, and star formation linked?
Over the next few years, JWST will likely identify new record-holders—but J0313–1806 will remain a cornerstone in our understanding of early supermassive black holes.
Frequently Asked Questions (FAQ)
Q: Is J0313–1806 the most distant object in the universe?
No. It is the most distant confirmed quasar, but galaxies like JADES-GS-z13-0 are even farther. However, quasars are much brighter, making them easier to study in detail despite the distance.
Q: How can a black hole grow so fast in such a short time?
That’s one of the key puzzles. Standard black hole formation via collapsing stars and steady accretion cannot explain its mass. This points toward models like direct collapse black holes or super-Eddington accretion.
Q: Will we find quasars even earlier than J0313–1806?
Yes—very likely. JWST and future telescopes will probe earlier cosmic epochs, potentially discovering quasars at z > 10, within the first 400 million years of the universe.
Q: What is the host galaxy of J0313–1806 like?
It appears to be a massive, dusty, star-forming galaxy, with signs of outflows driven by the quasar’s activity. It contains significant heavy elements—implying rapid early enrichment.
Q: What does this discovery mean for cosmology?
It challenges our current models of black hole growth, galaxy formation, and cosmic evolution. J0313–1806 is a crucial test case for understanding how the first massive structures emerged in the universe.
Final Thoughts – A Titan from the Dawn of Time
J0313–1806 is more than just a record-breaking quasar—it is a cosmic paradox. A billion-solar-mass black hole already in place just 670 million years after the Big Bang forces astronomers to rethink what is possible in the early universe.
Its discovery pushes the boundaries of:
Black hole formation physics
Galaxy–quasar coevolution
High-redshift observational techniques
As we uncover more quasars like J0313–1806, we may be forced to revise the timeline of structure formation itself.
For now, it stands as a beacon from the reionization era, offering a rare, powerful glimpse into the universe’s deepest past—and reminding us how much we still have to learn.